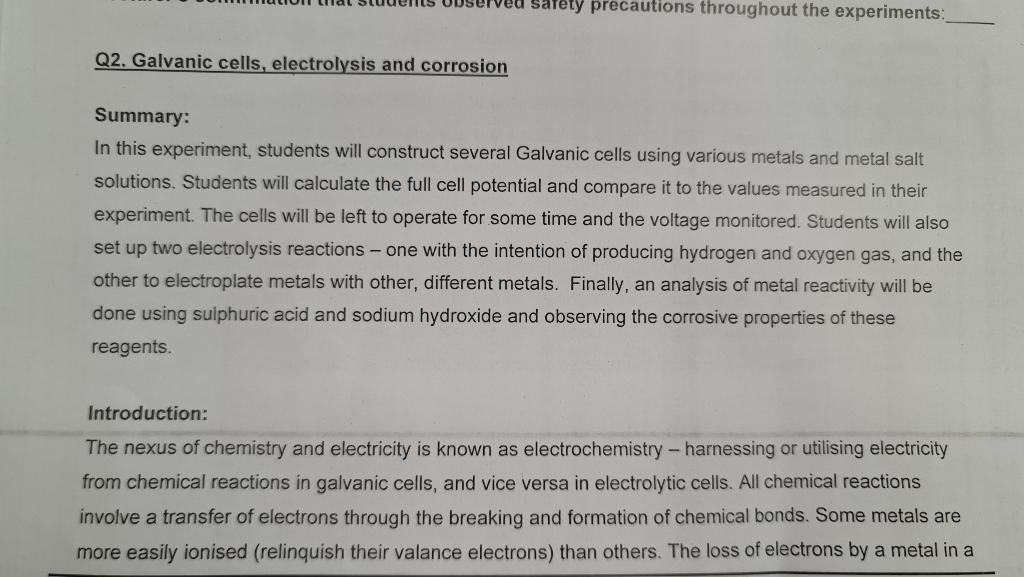
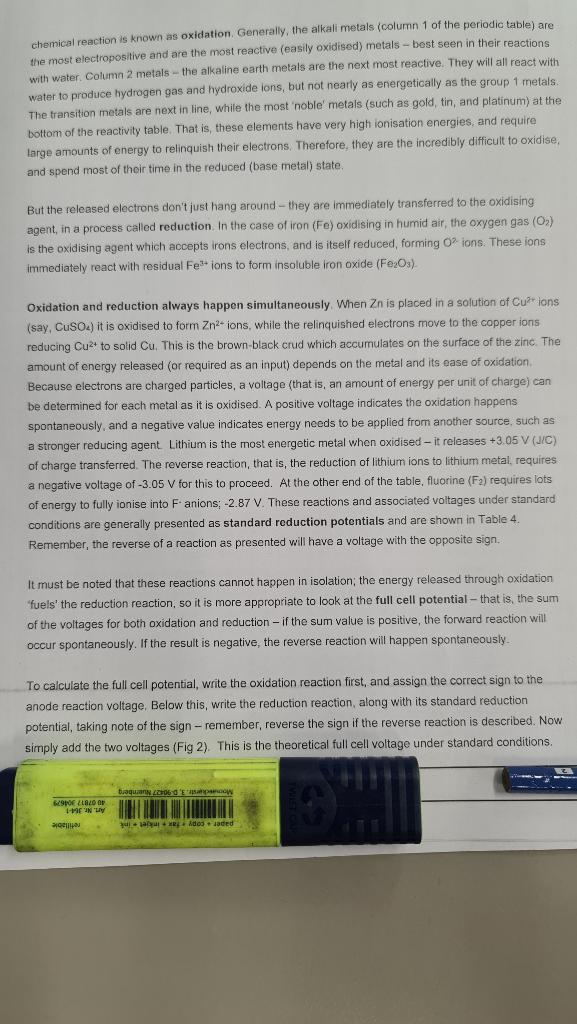
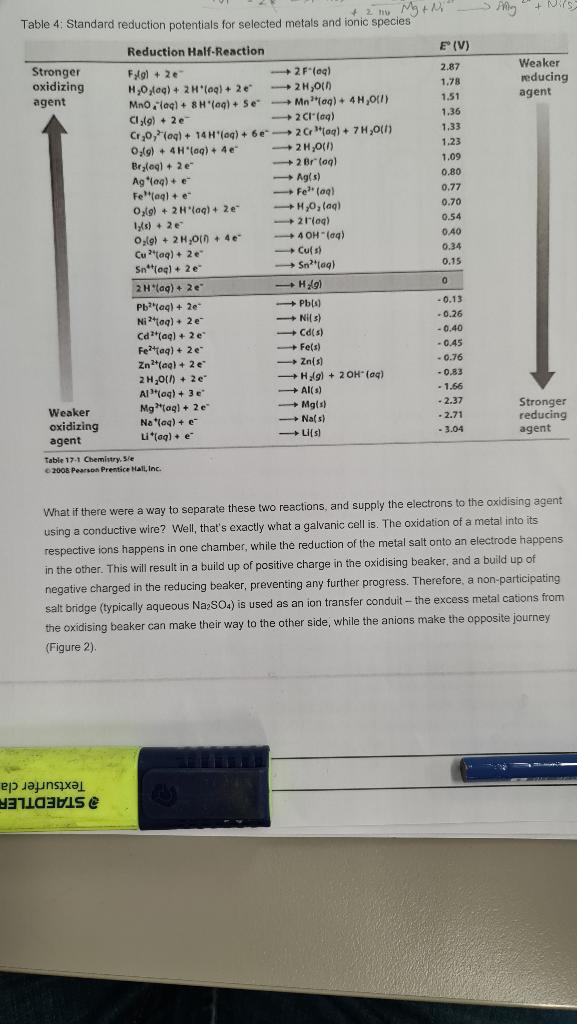
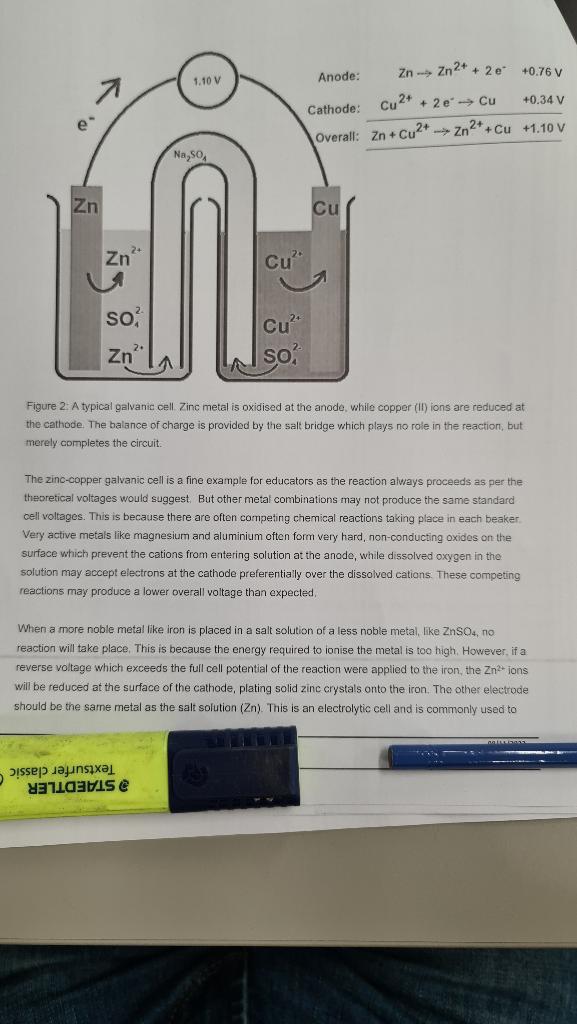
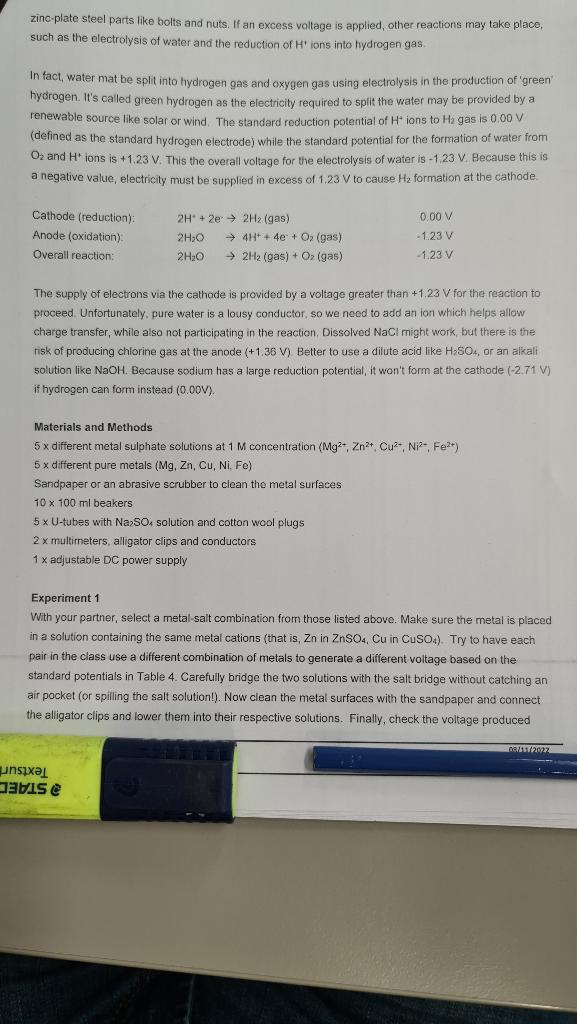
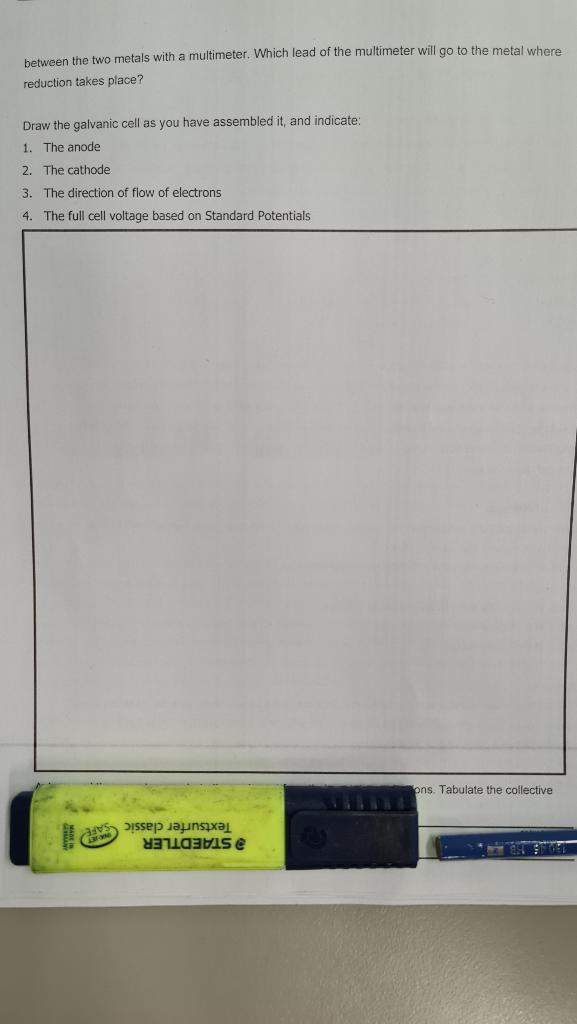
Q2. Galvanic cells, electrolysis and corrosion Summary: In this experiment, students will construct several Galvanic cells using various metals and metal salt solutions. Students will calculate the full cell potential and compare it to the values measured in their experiment. The cells will be left to operate for some time and the voltage monitored. Students will also set up two electrolysis reactions - one with the intention of producing hydrogen and oxygen gas, and the other to electroplate metals with other, different metals. Finally, an analysis of metal reactivity will be done using sulphuric acid and sodium hydroxide and observing the corrosive properties of these reagents. Introduction: The nexus of chemistry and electricity is known as electrochemistry - harnessing or utilising electricity from chemical reactions in galvanic cells, and vice versa in electrolytic cells. All chemical reactions involve a transfer of electrons through the breaking and formation of chemical bonds. Some metals are more easily ionised (relinquish their valance electrons) than others. The loss of electrons by a metal in a chemical reaction is known as oxidation. Generally, the alkali metals (column 1 of the periodic table) are the most electropositive and are the most reactive (easily oxidised) metals - best seen in their reactions with water. Column 2 metals - the alkaline earth metals are the next most reactive. They will all react with water to produce hydrogen gas and hydroxide ions, but not nearly as energetically as the group 1 metals. The transition metals are next in line, while the most 'noble' metals (such as gold, tin, and platinum) at the bottom of the reactivity table. That is, these elements have very high ionisation energies, and require large amounts of energy to relinquish their electrons. Therefore, they are the incredibly difficult to oxidise; and spend most of thoir time in the reduced (base metal) state. But the released electrons don't just hang around - they are immediately transferred to the oxidising agent, in a process called reduction. In the case of iron (Fe) oxidising in humid air, the oxygen gas ( O2) is the oxidising agent which accepts irons electrons, and is itself reduced, forming O? ions. These ions immediately react with residual Fe3+ ions to form insoluble iron oxide (Fe2O3). Oxidation and reduction always happen simultaneously. When Zn is placed in a solution of Cu? ions (say, CuSOL) it is oxidised to form Zn2+ ions, while the relinquished electrons move to the copper ions reducing Cu2+ to solid Cu. This is the brown-black crud which accumulates on the surface of the zinc. The amount of energy released (or required as an input) depends on the metal and its ease of oxidation. Because electrons are charged particles, a voltage (that is, an amount of energy per unit of chaige) can be determined for each metal as it is oxidised. A positive voltage indicates the oxidation happens spontaneously, and a negative value indicates energy needs to be applied from another source, such as a stronger reducing agent. Lithium is the most energetic metal when oxidised - it releases +3.05V(lC) of charge transferred. The reverse reaction, that is, the reduction of lithium ions to lithium metal, requires a negative voltage of 3.05V for this to proceed. At the other end of the table, fluorine (F2) requires lots of energy to fully ionise into F anions; 2.87V. These reactions and associated voltages under standard conditions are generally presented as standard reduction potentials and are shown in Table 4. Remember, the reverse of a reaction as presented will have a voltage with the opposite sign. It must be noted that these reactions cannot happen in isolation; the energy released through oxidation "fuels' the reduction reaction, so it is more appropriate to look at the full cell potential - that is, the sum of the voltages for both oxidation and reduction - if the sum value is positive, the forward reaction will occur spontaneously. If the result is negative, the reverse reaction will happen spontaneously. To calculate the full cell potential, write the oxidation reaction first, and assign the correct sign to the anode reaction voltage, Below this, write the reduction reaction, along with its standard reduction potential, taking note of the sign - remember, reverse the sign if the reverse reaction is described. Now simply add the two voltages (Fig 2). This is the theoretical full cell voltage under standard conditions. Table 4: Standard reduction potentials for selected metals and ionic species Table 17.1 Chemirtry, Sie o avos Pearson Prentice Hall, lnc. What if there were a way to separate these two reactions, and supply the electrons to the oxidising agent using a conductive wire? Well, that's exactly what a galvanic cell is. The oxidation of a metal into its respective ions happens in one chamber, while the reduction of the metal salt onto an electrode happens in the other. This will result in a build up of positive charge in the oxidising beaker, and a build up of negative charged in the reducing beaker, preventing any further progress. Therefore, a non-participating salt bridge (typically aqueous Na2SO4 ) is used as an ion transfer conduit - the excess metal cations from the oxidising beaker can make their way to the other side, while the anions make the opposite journey (Figure 2). Figure 2: A typical galvanic cell. Zine metal is oxidised at the anode, while copper (ii) ions are reduced at the cathode. The balance of charge is provided by the salt bridge which plays no role in the reaction, but merely completes the circuit. The zinc-copper galvanic cell is a fine example for educators as the reaction always proceeds as per the theoretical voltages would suggest. But other metal combinations may not produce the same standard cell voltages. This is because there are often competing chemical reactions taking place in each beaker. Very active metals like magnesium and aluminium often form very hard, non-conducting oxides on the surface which prevent the cations from entering solution at the anode, while dissolved oxygen in the solution may accept electrons at the cathode preferentialy over the dissolved cations. These competing reactions may produce a lower overall voltage than expected. When a more noble metal like iron is placed in a salt solution of a less noble metal, like ZnSO4, no reaction will take place. This is because the energy required to ionise the metal is too high, However, if a reverse voltage which exceeds the full cell potential of the reaction were applied to the iron, the Zn2+ jons will be reduced at the surface of the cathode, plating solid zinc crystals onto the iron. The othes electrode should be the same metal as the salt solution ( Zn ). This is an electrolytic cell and is commonly used to zino-plate steel parts like bolts and nuts. If an excess voltage is applied, other reactions may take place, such as the electrolysis of vater and the reduction of H+ions into hydrogen gas. In fact, water mat be split into hydrogen gas and oxygen gas using electrolysis in the production of 'green hydrogen. It's called green hydrogen as the electricity required to split the water may be provided by a renewable source like solar or wind. The standard roduction potential of H+ions to Ha gas is 0.00V (defined as the standard hydrogen electrode) while the standard potential for the formation of water from O2 and H+ions is +1,23V. This the overall voltage for the electrolysis of water is 1,23V, Because this is a negative value, electricity must be supplied in excess of 1.23V to cause H2 formation at the cathode. Cathode (reduction): Anode (oxidation): Overall reaction: 2H++2e2H2(gas)2H2O4H++4e+O2(gas)2H2O2H2(gas)+O2(gas) 0.00V 1.23V 1.23V The supply of electrons via the cathode is provided by a voltage greater than +1.23V for the reaction to proceed. Unfortunately, pure water is a lousy conductor, so we need to add an ion which helps allow charge transfer, while also not participating in the reaction. Dissolved NaCl might work, but there is the risk of producing chlorine gas at the anode ( +1.36V). Better to use a dilute acid like H2SO4, or an alkali solution like NaOH. Because sodium has a large reduction potential, it won't form at the cathode (2.71V) if hydrogen can form instead (0.00V). Materials and Methods 5x different metal sulphate solutions at 1M concentration (Mg2+,Zn2+,Cu2+,Ni2+,Fe2+) 5 different pure metals (Mg,Zn,Cu,Ni,Fe) Sandpaper or an abrasive scrubber to clean the metal surfaces 10100ml beakers 5 U-tubes with Na2SO4 solution and cotton wool plugs 2 muttimeters, alligator clips and conductors 1x adjustable DC power supply Experiment 1 With your partner, select a metal-salt combination from those listed above. Make sure the metal is placed in a solution containing the same metal cations (that is, Zn in ZnSO4,Cu in CuSO 4 ). Try to have each pair in the class use a different combination of metals to generate a different voltage based on the standard potentials in Table 4. Carefully bridge the two solutions with the salt bridge without catching an air pocket (or spilling the salt solution!). Now clean the metal surfaces with the sandpaper and connect the alligator clips and lower them into their respective solutions. Finally, check the voltage produced between the two metals with a multimeter. Which lead of the multimeter will go to the metal where reduction takes place? Draw the galvanic cell as you have assembled it, and indicate: 1. The anode 2. The cathode 3. The direction of flow of electrons 4. The full cell voltage based on Standard Potentials